Studying the Rapid Electrical Charging Behavior of Liquids
- ritambhara516
- 3 hours ago
- 3 min read
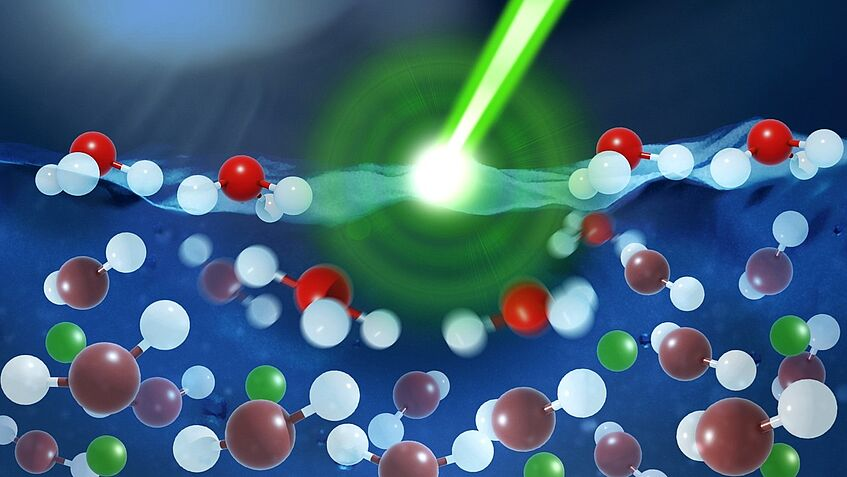
A new measurement method provides insights into the electrical charge of liquids at interfaces
When charged surfaces—such as battery electrodes or biological cell membranes—come into contact with liquids, they attract oppositely charged ions from the surrounding fluid. This interaction forms what is known as the electrical double layer, consisting of the charged surface and a layer of counter-ions in the liquid.
This structure is fundamental to the function of energy storage systems, but the speed at which it forms has long been difficult to measure. Now, a research team has developed a light-based method to observe this ultrafast process, confirming theoretical models and expanding their relevance to a wide range of systems—from biological membranes to advanced energy storage technologies.
Whether it's in electric vehicle batteries, where charge separation stores energy; in electrolytic capacitors, which are essential to nearly all electronic devices; or in water electrolysis to produce hydrogen and oxygen—these processes all involve the movement of charge carriers in liquid toward a surface or interface.
The same principle also plays a role in various biological mechanisms. In all cases, an electrical double layer forms at the interface: for example, at battery terminals, capacitor plates, electrolysis electrodes, or cell walls. One side of the interface, typically a solid surface like an electrode, holds a negative charge, while the liquid side accumulates positive ions in response.
Understanding how fast these extremely thin layers—just a few nanometers in thickness—can form or respond to changes is crucial. This insight directly affects how quickly energy storage devices can absorb and release electrical energy, a key factor for improving performance in applications like rapid battery charging.
Limitations of previous models
When only a small number of mobile charge carriers are present, theoretical models and experimental data have reliably explained the ion dynamics within the electrical double layer. However, in systems with higher concentrations of charge carriers—such as biological environments or battery technologies—these models no longer hold true, and the precise mechanisms of double layer formation have remained unclear.
“Up until now, we haven’t been able to fully observe the detailed processes behind the formation of the electrical double layer,” explains Mischa Bonn, Director at the Max Planck Institute for Polymer Research. “Electronic circuits simply lack the temporal resolution to capture the extremely fast motion of ions. To overcome this limitation, we turn to ultrafast optical techniques.”
Optical measurement method offers new possibilities
To investigate how electrical double layers form, researchers from the Max Planck Institute for Polymer Research and the University of Vienna employed an optical measurement technique. They introduced acid into water, generating positively charged ions (H₃O⁺), which naturally migrate to the water's surface and form an electrical double layer.
“We used a strong infrared laser pulse to heat the surface and displace H₃O⁺ ions, thereby disrupting the double layer,” explained Ellen Backus, a physical chemistry professor at the University of Vienna. “By following up with additional laser pulses after a controlled delay and analyzing the reflected light, we could track how the ions moved away from the surface and how the system returned to equilibrium.”
To deepen their understanding, the team also ran computer simulations alongside their experiments. This combined approach confirmed that electric fields are the main drivers of double layer formation—even at high ion concentrations.
Their findings, published in the journal Science, introduce a powerful new method for exploring interfacial processes in both chemical and biological systems. The study also reveals that even complex surface interactions can often be described using relatively straightforward physical models, validating the accuracy of existing theoretical frameworks.
Comments